Sign up for daily news updates from CleanTechnica on email. Or follow us on Google News!
In recent months I’ve returned to the overlapping subjects of geoengineering and carbon capture, both of which I’d mostly satisfied myself about years ago. Both, however, are re-ascending the hype scale and at least for aspects of geoengineering, my curiosity was unsatisfied.
The most recent news was that Germany’s vice chancellor, Robert Habeck, has publicly backed a significant carbon capture and sequestration plan as actual decarbonization of the economy is not on track for targets. What’s notable about this is that he’s a German Green Party politician who is also the economy and climate minister. That’s a lot of interesting hats for one person to wear. He asserts that the technology is now mature and safe in a fair amount of divergence from empirical reality.
One assumes no one has bothered to tell him about Satartia, Mississippi’s, travails a small handful of years ago. That tiny town of 41 in a county with a population density orders of magnitude smaller than any populated part of Europe, saw a carbon dioxide pipeline break outside of town, 1.6 kilometers away, in fact. The liquid carbon dioxide expanded by 590 times, and as it was heavier than the air we breathe, it rolled downhill into town. It rendered many people unconscious and in convulsions before it diffused into the atmosphere. Dozens were treated in hospital, from a town that only had 41 inhabitants. A couple of hundred people from the surrounding area were evacuated. Emergency vehicles with internal combustion engines didn’t work. Hours later, dangerous levels of carbon dioxide still remained in many homes and declivities.
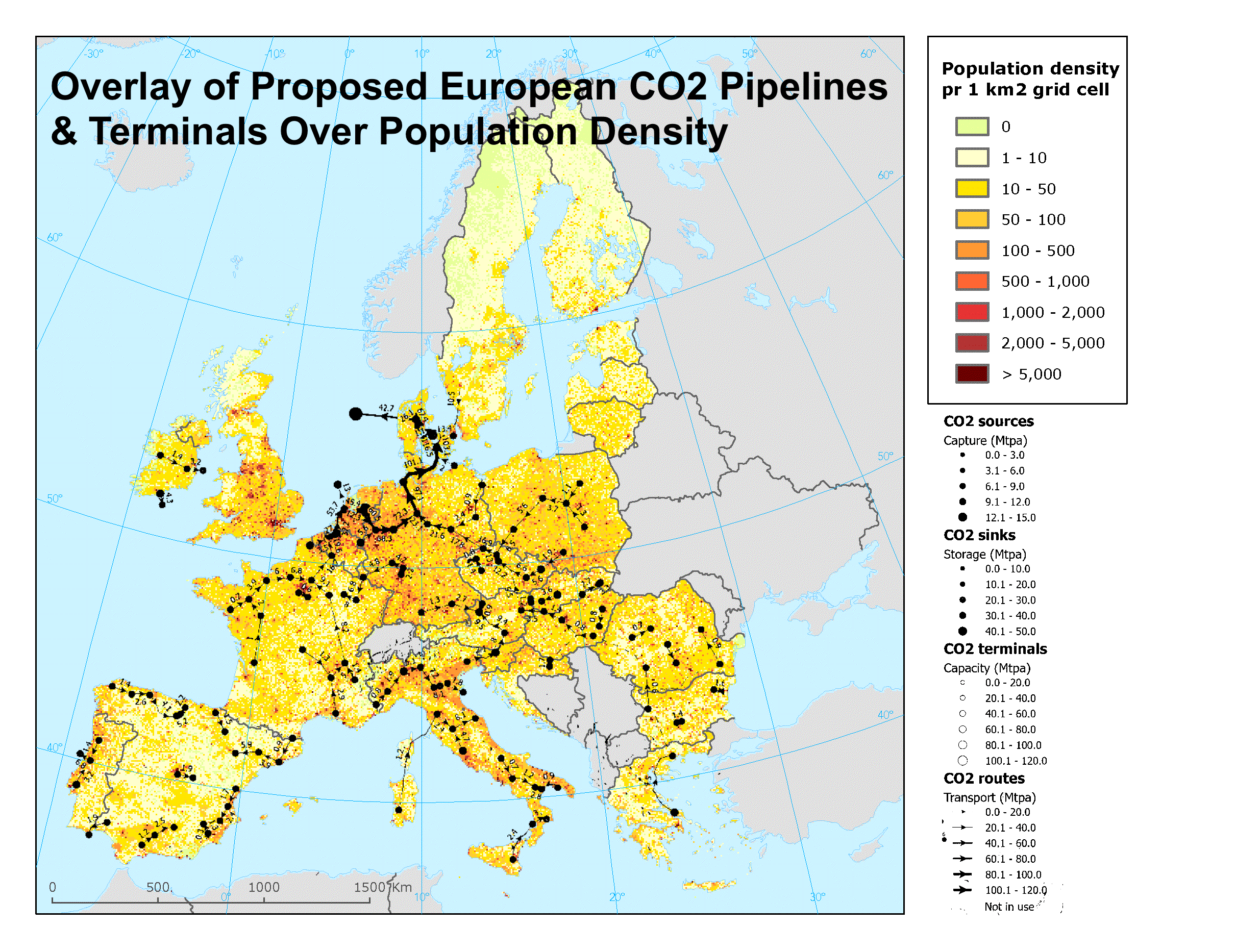
Recently, I reviewed an almost hundred-page report assessing European carbon dioxide pipelines, sinks, and terminals options. The report said absolutely nothing about safety, health risks, or what might happen if a carbon dioxide pipeline burst. Apparently you have to know basic chemistry and biology to realize that carbon dioxide blankets the ground, pools in low-lying areas and homes, and asphyxiates people and animals. It’s really basic chemistry and biology, by the way.
During that effort I created the graphic above, overlaying one of the proposed sets of carbon dioxide movement infrastructure over a population density map of Europe. Notice how all of those pipelines seem to run through the more heavily populated parts of Europe? Remember that Satartia barely exists yet had dozens unconscious and hospitalized? Seeing a problem here?
Also recently I was asked my opinion of putting milk of magnesia, aka magnesium hydroxide, into sea water to enhance the ocean’s ability to absorb more carbon dioxide. I was more aware of the implications for shellfish of increased carbon dioxide in the atmosphere being absorbed by the oceans, that it was hindering formation of crustacean and mollusk shells.

I knew that I had to return to this after looking at geoengineering years ago, finding in general that if we had to use solar geoengineering, that ugly bandaid that staunched a wound even as more wounds were inflicted, we would have lost, but that ocean geoengineering might be necessary.
And so with the question I returned to the problem and started to both understand the problem and the proposed solutions better. Naturally, I wrote about it. Every molecule of carbon dioxide that enters the oceans takes another molecule of carbon bound into a carbonate ion out of the reach of shellfish by moving the carbon in both the carbon dioxide and the carbonate ion into two carbonate ions. And the more carbon dioxide that entered the water, the less carbon dioxide could be absorbed by the water as it alkalinized. That’s a two-edged sword.
The milk of magnesium people were trying to block the process by forming magnesium carbonate instead, making the ocean a bit more alkaline and leaving more carbon atoms for the shellfish. That would enable the ocean to absorb more carbon dioxide, requiring more magnesium hydroxide, but it would, chemically, work. Unfortunately milk of magnesium at industrial scales costs hundreds of dollars per ton and has a carbon debt for manufacturing higher than the extra carbon dioxide drawdown. Not really a solution and the firm that won the Musk et al. Carbon Drawdown X Prize with its two pilot plants is trying desperately to pivot to something that actually makes any sense, although they really don’t want to admit it.
What are they pivoting to? Electrochemical separation of highly alkaline mining slag. You know, the stuff that’s full of a stew of toxic chemicals that we really try to prevent getting back into the ecosystem. If they could actually do what they are asserting they will be able to do, they would be vastly better off selling the products, as they are highly valuable and currently have big carbon debts of their own. I’m skeptical.
This isn’t to cast doubt on electrochemistry in general, by the way. It’s an astounding technology and all of those amazing batteries powering our electric vehicles, iPhones, and watches depend on it, as does making aluminum and even plating metals. I was a strategic advisor to an electrochemistry flow battery firm for a couple of years and still have high hopes for them.
But a lot of people are asserting magical electrochemical solutions from stews of complex molecules and there are a lot of risks inherent in the space.
Chip in a few dollars a month to help support independent cleantech coverage that helps to accelerate the cleantech revolution!
Enter Captura, a California carbon capture firm founded out of the California Institute of Technology, one which has managed to raise over $33 million dollars for its alternative approach to enhancing oceanic carbon dioxide uptake. What’s the solution? Pump lots and lots of sea water out of the ocean into their technology. Separate a bit out then split it into more and less acidic portions with an electrodialysis system with their proprietary membrane in it. Mix the acidic bit back into the rest of the water. That reacts with bicarbonate ions — my assumption as they are 90% of the carbon in the oceans — and turns them into carbon dioxide. Filter the carbon dioxide out. Add the less acidic portion of water back in to return the alkalinity to normal sea water. Return the sea water to the ocean. Find something to do with the carbon dioxide.
Will this work? Of course, up to a point. It’s fairly basic electrochemistry working on well understood organic chemicals. I’m sure in the lab with biocarbonate ions in brine it works spectacularly. Unfortunately, the lab is not the real world, and I see many red flags that make me think this won’t work.
First off, sea water is full of life, salts, and minerals, and those things cling to everything they touch. That’s why ship defouling is such a problem. Fouling of a Captura plant is a big concern. Desalination plants obviously manage this, but they are working to remove everything except water, not a tiny percentage of specific molecules. The extra things are often bigger than CO2 molecules as well, making the filters challenging. CO2 can sit in dissolved suspension without bubbling to the surface immediately. That is certainly viable in a simple brine with carbonate ions, but more difficult in real sea water with highly variable constituents.
The electrodialysis system is concerning in this regard as it has a proprietary membrane technology. Do you know what the most expensive part of a lot of batteries is by mass? The membrane. Ultrathin, ultratough membranes that allow ions to pass through them while blocking everything else are expensive. They tend to like very pure things on either side of them, which is fine inside sealed batteries, but is a persistent problem for both electrolyzers and fuel cells where water, air, and hydrogen have to be very clean and within precise parameters.
Sea water is a lot less pure than fresh water. Those membranes are going to be taking a beating and they aren’t commoditized membranes, they are lab membranes, it appears. That means that they are going to be much more expensive and much more challenging to scale up to any reasonable size. Even then, they’ll require regular replacement.
Desalination makes economic sense where 2.5x the cost of water is required because 95% of what passes through the system is the product, fresh water, and only about 5% is waste that’s returned to the ocean. Even there, very salty brine pouring back into the ocean is a big concern that must be managed carefully.
But in this case, the carbon content of the ocean is lower than the carbon content of atmosphere, under 300 parts per million or 0.03% as I understand it. That means they will have to pump about 3,333 tons of seawater, i.e. 3,333 cubic meters, to get a ton of CO2. As long as you aren’t moving the water too far uphill, the energy requirements aren’t absurd, but to get a barely material million tons of carbon dioxide they’d have to move 3.3 billion tons of water.
For context, that’s 22 days of all of the United States’ freshwater withdrawals from the Great Lakes. That’s about 3.5 times as much water as the Grand Coulee Dam can hold when it’s full. That would require moving nine million tons of water every day. That’s about 2,000 Olympic swimming pools full of water every day. That’s a lot of water.
Even assuming that the water is only being raised five meters from ocean level to pass through the system, that’s a lot of mass being moved up five meters. My napkin math suggests that this would take about 45 GWh a year. That’s enough to power about four million homes for a year. To extract a million tons of carbon dioxide from the atmosphere.
Why not just use the renewable electricity to power homes, heat pumps, cars, and industrial heat, eliminating a lot more emissions instead? At the US average grid electricity carbon intensity of about 387 kg CO2e/MWh — hey, fun fact, China’s electricity grid carbon intensity has plummeted to only 550 kg CO2e/MWh and is rapidly declining further — using the electricity directly would avoid over 17 million tons of carbon dioxide or equivalent. As always with capturing diffuse amounts of carbon dioxide out of the atmosphere or oceans after we’ve stupidly released it, reversing entropy is a lot less efficient than avoiding it.
And that’s with a system that approaches 100% efficiency in turning bicarbonate ions into carbon dioxide and capturing it. The likely efficiency will be much lower. I’d be surprised if they made it to 50%, so 34 times worse than just using the low-carbon electricity. But let’s pretend it’s 100% efficient.
Then there’s the problem of diffusion, the process by which things spread out fairly evenly through gases and liquids. Water moves and things diffuse in it, but more slowly than in air. Capture would be removing bicarbonates locally. That means that they are lowering the parts per million locally and getting diminishing returns. There will be some optimal point, but it’s going to be likely below 200 ppm, so call it 5,000 cubic meters of water to get a ton of CO2. Probably they will have to restrict their sites to places where there are lateral currents alway bringing new sea water into the system.
Then there’s that big problem of ocean acidification making carbon atoms inaccessible to shellfish, so they can’t form their shells. Excess CO2 uptake does that as noted above, but so does the Captura process it appears. Clearly, environmental assessments are going to be concerned about elimination of shellfish mineral requirements. Got a dead ocean somewhere without shellfish? So in addition to places with regular lateral currents, the sites also have to have no significant populations of crustaceans or mollusks down-current.
Finally, there’s the big question of what they are going to do with the carbon dioxide. Sequestration sites aren’t magically beside sites that happen to have lateral currents, very low shellfish concentrations, and good renewable connections. CO2 pipelines have their own problems, as I pointed out earlier. Carbon dioxide pipelines are going to leave this million tons per year site to go somewhere and the safety requirements means it has to be basically straight into the water to offshore sequestration sites.
So, the technology can only be sited beside the ocean on relatively low shorelines where there are strong lateral currents to keep bringing bicarbonate ion-rich ocean water into the intakes where there are few shellfish down-current where there is a lot of renewable electricity to run the process and there are good carbon sequestration sites reasonably close to shore.
And even then it will waste a lot of low carbon electricity that could have been preventing a lot more carbon emissions. Personally, I’m completely unsurprised that this napkin math shows that Captura’s process is completely non-viable compared to alternatives until the rest of the world’s economy is approaching 100% carbon neutral. As I said about Carbon Engineering, great, you’ve proved it works, now put it on the shelf until after 2050.
Have a tip for CleanTechnica? Want to advertise? Want to suggest a guest for our CleanTech Talk podcast? Contact us here.
Latest CleanTechnica TV Video
CleanTechnica uses affiliate links. See our policy here.